In case you haven’t heard, China’s first space station, Tiangong-1 is about to make an uncontrolled re-entry into Earth’s atmosphere. That’s an 18,750 pound (8,500 kg) chunk of stuff, about to fall in a blaze of glory. We don’t know when, and we don’t know where…. but probably some time in March, 2018.
To understand why, I’ll have to back up and explain a few things.
The dynamics of a body in orbit are very interesting, in that they seem perpetual, and timeless – the clockwork of the solar system. The truth is, objects in orbit around other objects, whether those be artificial satellites, moons, planets, comets, etc. are subject to all kinds of external forces, where gravity just happens to be the most significant.
What is “orbit”, exactly? Well, objects in Earth orbit are actually falling – constantly accelerating toward the planet – they’re just moving too fast to hit it. The “weightlessness” that astronauts feel is not an effect of being in space, it’s the result of being in orbit. Astronauts are in constant free-fall, but moving so fast that the thing they’re falling toward (Earth) is constantly slipping out of the way.
Take Issac Newton’s cannonball thought experiment. If you drop a ball, it will fall straight down and hit the ground. If you throw it horizontally, it will take about the same time to hit the ground (because its acceleration downward is the same as just dropping it), but it will hit the ground farther away.
Now suppose you had a cannon powerful enough to fire that ball over the horizon. Gravity will pull the ball down, but it will have moved so far, so fast, that the Earth has literally curved away from where it was at the ball’s starting point, and the ball will take longer to hit the ground. Fire your ball faster and faster, and eventually it will move so fast that the Earth drops out from under it at the same rate it is falling, and it will never hit the ground again…. voila, and congratulations, you’ve achieved orbit! Fire even faster (about 25,000 mph, 11.2 km/s or Mach 33, at the surface), and you’ll be moving so fast that you’ll escape Earth’s gravity completely, and fly off into space. Anything between the speed necessary to just barely come back and hit your own cannon (i.e. circular orbit) and escape velocity will still achieve AN orbit, but faster velocities will create more elongated elliptical orbits that go farther into space before slowing and falling back to round Earth and hit your cannon again (the more elongated, the more eccentric the orbit).
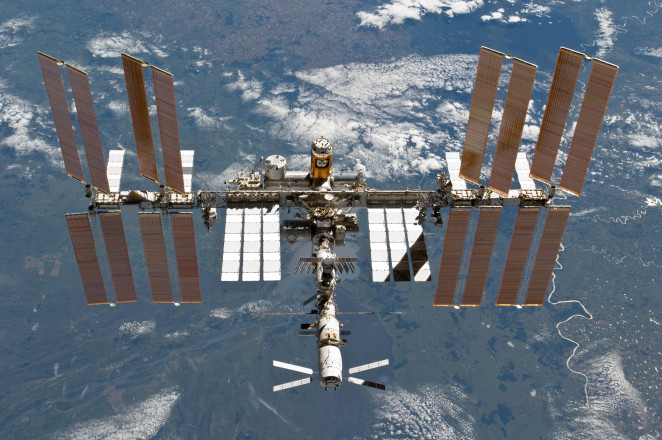
The International Space Station; NASA
Getting things into orbit, then, is not as easy as just getting them “high”, you also have to get them to go fast. Without getting too much into the math, it’s important to note that things in lower orbits require more speed to stay there than things in higher orbits. Low Earth Orbit (LEO) satellites, like the International Space Station, circle the Earth once every 90 minutes or so, at 17,150 miles per hour, at an altitude of roughly 250 miles. Go high enough, and you can slow that to one orbit every 24 hours. That’s only 6,900 mph (roughly), but a whopping 22,236 miles up. At this altitude, and ONLY at this altitude, you can park a satellite over the equator and exactly match Earth’s rotational velocity – which is exactly what geostationary satellites, like many communications and weather observing satellites, do.
(If you care about SOME of the math, acceleration due to gravity is a function of 1/r^2, while acceleration to maintain a circular orbit is a function of V^2/r, where V is your speed – and you need these things to balance. Conveniently the mass of the object in orbit drops out of both sides of the equation, and is irrelevant – only speed and altitude matter. So large space stations and tiny resupply modules can rendezvous quite nicely, matching speeds at the same altitude.)
Orbits are also, typically, tilted. Launching due east from wherever you start takes advantage of the extra speed of the Earth’s surface at the launch site – essentially giving you a “push” that is maximized at the equator, so launching from near the equator is most efficient. Launching from Kennedy Space Center, due east, you will get some assistance from the rotation, but will start your journey at 28.5 degrees north. A circular orbit will be, therefore, tilted off the equator by 28.5 degrees. (You can’t stay at 28.5 north and still orbit the center of the Earth, so your launch site will, largely, define your starting tilt). In the case of the ISS, the tilt actually has to be higher – 51.6 degrees. This is to accommodate rendezvous with vehicles launched from Baikonur Cosmodrome, Russia’s main launch site (which is actually in Kazakhstan), situated at 45.6 degrees latitude. Given this location, launches from Baikonur COULD achieve a 45.6 degree tilt, but doing so would drop spent rocket stages over China. SO, the Russians crank the orbit a little north of east, and the result is a 51.6 degree tilt, minimum. ISS maintains this orbit for mission convenience, just as its altitude is also adjusted to comply with its resupply modules. In some ways, this just works out. A tilted orbit at 51.6 degrees will take advantage of the Earth’s rotation underneath it so that the ISS follows a sinusoidal path over the surface. Slightly out of phase with the rotation, this means the ISS will, eventually, pass over every location on Earth, at some point, up to plus/minus 51.6 degrees latitude.
Orbit selection is therefore driven by launch site, mission parameters, weight, fuel, and a tradeoff between height, and speed, all with a focus on minimizing energy in the form of rocket fuel. Unless you NEED to be geostationary, or are leaving Earth entirely, there’s really no point in going any farther from Earth than you have to, so Low Earth Orbits (LEOs) are popular. Once there, there are other considerations – like in the case of the ISS, you need resupply. In that case, your orbit needs to be low enough that supply vehicles (like Soyuz, Progress, the SpaceX Dragon, or once upon a time, the Space Shuttles) can reach and dock with you. Space Shuttles, heavy as they were, were a limiting factor — back in the days when they visited, the ISS had to cruise a little faster, and a little lower, at about 220 miles. Now that the Shuttles are no longer making the trip, the ISS can be a little higher – around 250 miles.
That difference is important, because, though 250 miles is comfortably in space (generally agreed to be anything more than 99 miles up), there’s still stuff up there, and there’s more stuff at 220 miles than at 250. Not a LOT of stuff in either case – residual atmosphere, stuff from coronal mass ejections – but enough that the effect of interacting with incredibly sparse molecules is enough to slowly but surely slow the ISS – and other satellites – down. And when they slow, they start to fall.
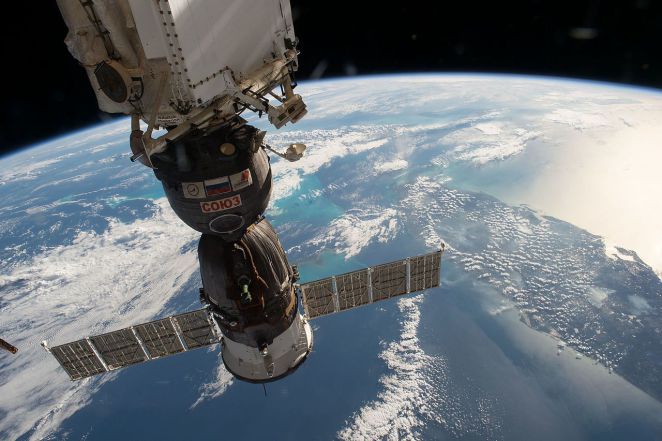
Soyuz docked to ISS over Florida; NASA
But wait, didn’t I just say that slower orbits were stable at higher altitudes? Yes, I did – the important word there being “stable”. Changing orbits requires two maneuvers, and the most efficient idealized case of this (going from one circular orbit to another) is called the Hohmann Transfer, named for the astrodynamicist that worked it out.
To explain the orbital transfer, consider a resupply mission to the ISS, where you have to achieve rendezvous from a ground-based launch. A vehicle on the launch pad is given a launch window that places the launch site in the same orbital plane as the target vehicle, the ISS, in this case. We sit on the pad, waiting for the orbital plane of the ISS, following its sine wave over the planet, to line up with our location. When it does, we have a viable launch window, and we blast off into space in the same plane as the target. We roll east to take advantage of the eastern velocity our launch site already has, due to rotation of the planet, turning north or south as necessary to match the ISS direction on that pass, and then settle into an orbit below and behind the ISS – but faster. We can afford to coast here for a while, but not too long, because the station needs those supplies – however, the planar alignment is more important, generally, than specific position of the station during the launch. As we near the station, catching up from below, we have to do two velocity changes to match orbits with the station. If we were to use a Hohmann transfer, we’d first accelerate along the direction of travel, giving the resupply module a positive delta-V (short for “change in velocity”). This has the effect of making our orbit non-circular, and more elliptical, with the perigee (closest point to Earth) matching our lower orbit, and, if done right, the apogee (farthest point from Earth) just barely grazing the orbit of the ISS, very near the ISS itself. After this initial delta-V, we coast upward toward the station – and because we’re going upward, we start to slow (just like a baseball at the top of its arc). When we reach apogee, and the height of the ISS, we’ve actually slowed to the point that we will start to fall back and follow our elliptical path back toward the lower altitude (perigee), unless we do something – so a second burn is initiated to give us another delta-V. This brings our speed up to the speed that matches that required for a circular orbit at this new altitude – in effect raising the perigee to the same height as the apogee and re-circularizing the orbit. So even though our resulting velocity is SLOWER than where we started, we had to SPEED UP TWICE to get here. And now we can do the fine tuning required to dock with the ISS.
Dropping altitude is similar – you have to slow down first, let the altitude drop, during which you will accelerate, falling toward the planet, and then slow down again to circularize the orbit at the new altitude. Slow down twice to wind up going faster…
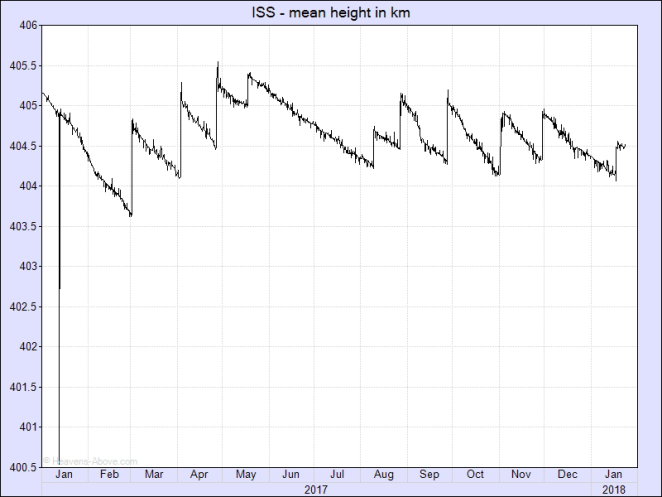
Mean height of ISS in km. Near-vertical lines show orbital adjustments, either from onboard thrusters or help from docked supply modules. Source: Heavens-above.com
Once we have docked with the ISS, we can offload supplies, and fuel for the Zarya and Zvezda modules. While there, the resupply module can also fire its thrusters, and push the ISS, adding a little delta-V to push its orbit a tad higher. This is done every month, on average – a little less frequently now that the ISS is at 250 miles – because without this adjustment, the drag caused by the infinitesimal amounts of stuff cause the ISS to slip lower and lower. If no supply ship is docked to give a shove, the thrusters on Zarya and Zvezda can do the job. But if it isn’t done regularly, the ISS will come down.
Which brings us back to Tiangong-1.
Launched in 2011, the station (whose name translates to “Heavenly Palace”) was used to test autonomous docking and other studies before being occupied in 2012. The last Chinese astronauts left it in 2013, but rather than de-orbit the station, it was left in place to monitor endurance and lifespan of certain components, and as a backup for the slightly larger Tiangong-2, which launched in 2016. (Tiangong-2 is another testbed – the final station, Tiangong-3, is expected to be operational in 2022).
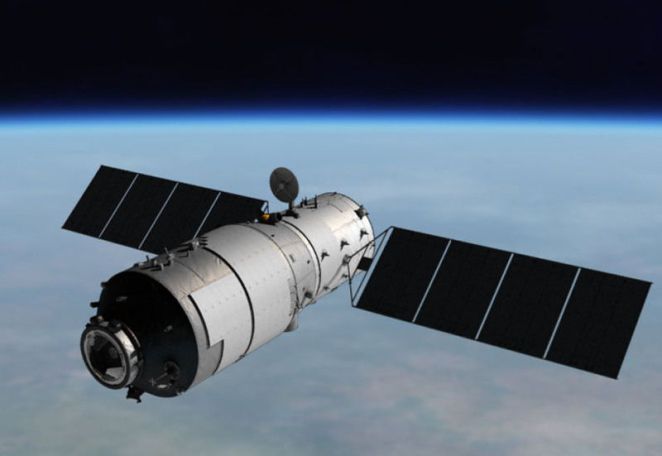
Artist’s Conception of Tiangong-1; Credit: China Manned Space Engineering (CMSE)
By early 2016, however, Tiangong-1’s orbit had begun to decay – at a rate of about 10km (6 mi) per month. The Chinese National Space Agency ultimately confirmed that the station had lost power, and was in an uncontrolled descent. In November, 2017, the rate of descent increased sharply, and as of January 2018, the altitude is only about 180 miles.
At some point, in the near future (Mid March, plus or minus a couple weeks), the drag will be so great that the station will slow significantly, be gripped by the atmosphere, and make its final plunge, likely burning up before any pieces reach the ground. Events like this have happened before – with the US Skylab (ten times the size of Tiangong-1) in 1979, and with Russia’s Mir in 2001. If you want to see it before it comes down, check out Heavens-Above. This site has a dedicated tracker showing visible passes of the doomed spacecraft – just make sure you’ve set your location.
So while it might be a bit embarrassing for the Heavenly Palace to come down out of control, instead of on a planned re-entry, it’s not a reason to worry. It’s just what happens, if you don’t CONSTANTLY work to maintain a precise orbit.
Get Out There!
Troy
http://www.flying-squirrel.org